"Discover the cutting-edge technology of quantum computing and its potential impact on the field of drug discovery. From simulating complex molecular interactions to accelerating the drug development process, quantum computing is revolutionizing the way we approach the search for new and effective treatments. Learn about the latest research and developments in this exciting field, and discover the potential of quantum computing to revolutionize the future of medicine."
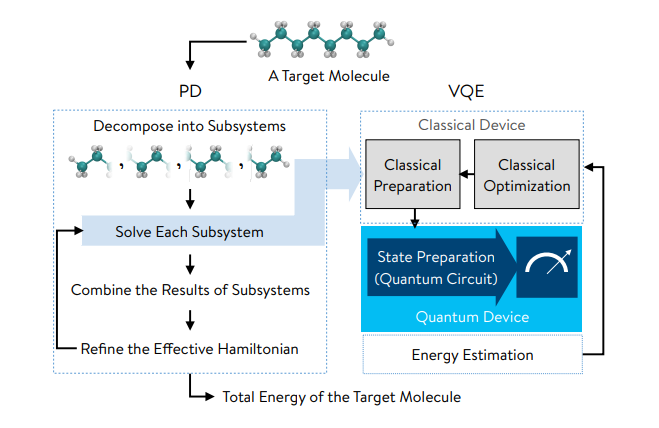
Drug discovery is a complex and time-consuming process that involves identifying and developing new compounds that can be used as therapeutics. The process typically involves several stages, including target identification, lead discovery, lead optimization, preclinical testing, and clinical trials. Each stage is associated with a set of computational and experimental challenges that can slow down the discovery process. Quantum computing has the potential to speed up the drug discovery process by providing new tools for solving complex computational problems.
For example, quantum computing can be used to perform large-scale molecular dynamics simulations, which are used to study the behavior of biomolecules such as proteins and nucleic acids. These simulations can provide valuable information about the structure and function of biomolecules, which can aid in the discovery of new drug targets.
Another area where quantum computing can be used to speed up drug discovery is in the design of new compounds. In this context, quantum computing can be used to perform quantum chemistry calculations, which can provide detailed information about the electronic structure and reactivity of molecules. This information can be used to design new compounds with specific properties, such as high binding affinity for a particular target.
Quantum computing can also be used in drug repurposing, where existing drugs are used to treat new diseases. Classical computer algorithms are limited in their ability to search through the large chemical space of existing drugs. Quantum computing can be used to perform large-scale simulations to find new drug candidates with high binding affinities to new targets.
There is a growing body of research that demonstrates the potential of quantum computing to speed up drug discovery. For example, a research team at the University of Southern California used quantum computing to screen a library of over 1 billion compounds in search of potential candidates for treating COVID-19. The team used a quantum algorithm to evaluate the binding of each compound to the spike protein of the SARS-CoV-2 virus and identified several compounds that have high binding affinities.
Another example, a team at the University of Waterloo and 1QBit, a quantum computing software and consulting firm, used quantum annealing to speed up the discovery of new drugs. The team used a quantum annealer to search through a library of over 1 million compounds in search of those that bind to a protein associated with cancer. They found that the quantum annealer was able to search through the library much faster than a classical computer and identified several compounds that have high binding affinities to the protein.
In conclusion, quantum computing has the potential to speed up the drug discovery process by providing new tools for solving complex computational problems. From large-scale molecular dynamics simulations, quantum chemistry calculations to drug repurposing, quantum computing can be used to perform large-scale simulations, identify new drug candidates, and design new compounds with specific properties. While the technology is still in its infancy, research teams are already demonstrating the potential of quantum computing to speed up drug discovery and pave the way for new treatments for a wide range of diseases.
Molecular dynamics (MD) simulations are computer-based simulations that use mathematical models to study the motion and interactions of atoms and molecules. These simulations can provide detailed information about the structure, dynamics, and thermodynamics of biomolecules such as proteins, nucleic acids, and lipids. The simulations are based on the laws of classical mechanics and quantum mechanics, and they take into account the forces acting on the atoms and molecules, such as electrostatic forces, van der Waals forces, and hydrogen bonding.
MD simulations typically involve the integration of Newton's equations of motion for each atom in the system, using a suitable force field to calculate the interactions between atoms. This allows the simulation to predict the time evolution of the system, which can provide information about the structural changes, conformational changes, and thermodynamic properties of the biomolecules. MD simulations are widely used in the field of biochemistry and structural biology to study the properties of biomolecules, such as protein folding, ligand binding, and enzyme activity. They can also be used to study the interactions between biomolecules and drugs, and to predict the effects of mutations on protein structure and function. Additionally, they can be used in the field of material science to simulate the behavior of various materials under different conditions. One of the main advantages of MD simulations is that they can provide detailed information about the atomic-level mechanisms of biological processes that is difficult or impossible to obtain experimentally. However, MD simulations can also be computationally demanding, and they typically require large amounts of computational resources and high-performance computing. It's worth noting that in order to obtain accurate and reliable results, MD simulations require highly accurate models, and therefore require a large amount of experimental data as input, such as crystal structures, NMR data and so on.
Download PDF
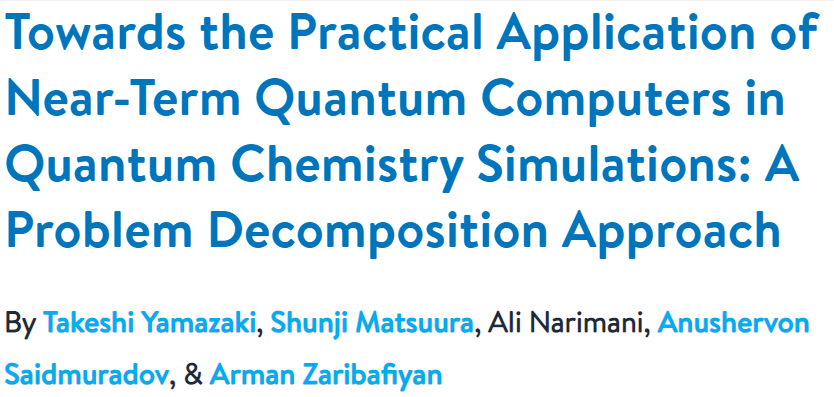

Comments